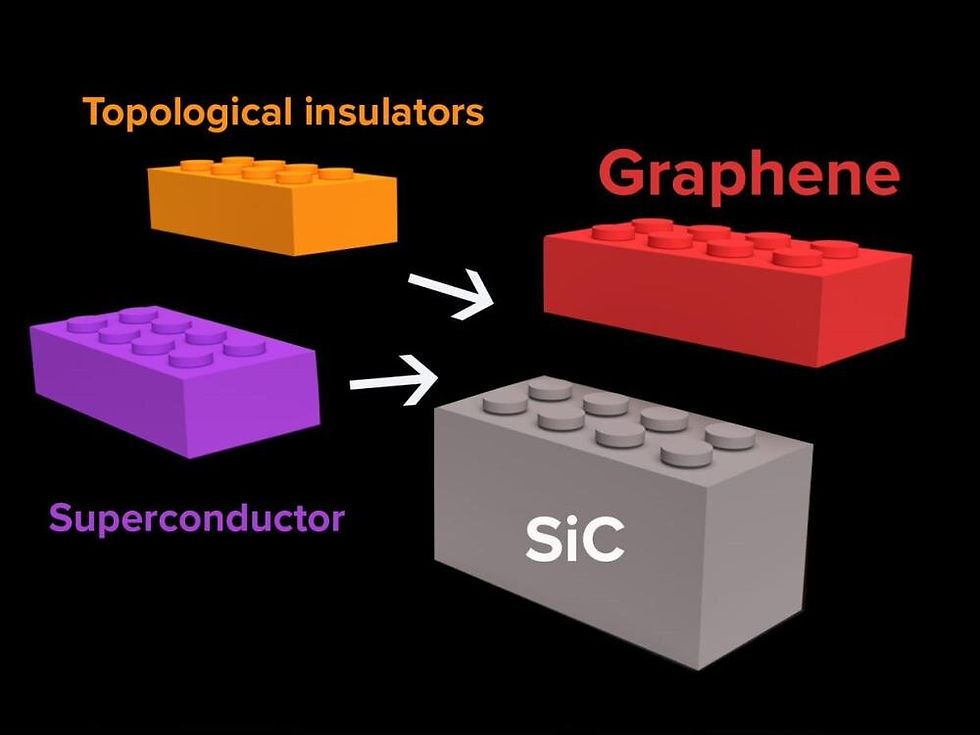
A new form of heterostructure of layered two-dimensional materials may overcome the key barriers in quantum computing, which is a rapidly developing field of computer science. This article delves into the details of the research conducted by an international team of researchers at Penn State Center for Nanoscale Science, who have demonstrated that their development of heterostructure may enable the realization of a topological superconductor, which is necessary for building topological qubits. The article also explains the differences between classical and quantum computers and the issues associated with building a quantum computer, and how topological qubits may offer a solution to the problem. Furthermore, the researchers have used a synthesis technique known as confinement heteroepitaxy, which involves inserting a layer of epitaxial graphene to overcome the challenges of creating heterostructures. This article explores the implications of the research and its potential future applications in building a topological quantum computer.
Table of Contents:
I. Introduction
VII. Research Findings
IX. Conclusion
I. Introduction:
The field of computer science has been revolutionized by the development of quantum computing, which has the potential to solve complex problems that classical computers cannot. However, there are several challenges associated with building a quantum computer. An international team of researchers at the Penn State Center for Nanoscale Science has developed a new form of heterostructure of layered two-dimensional materials that may overcome these challenges and enable the realization of a topological superconductor, which is necessary for building topological qubits.
II. Classical and Quantum Computers:
A classical computer consists of billions of transistors, known as bits, that are governed by binary code ("0" = off and "1" = on). A quantum bit, also known as a qubit, is based on quantum mechanics and can be both a "0" and a "1" at the same time. This is known as superposition and can enable quantum computers to be more powerful than regular, classical computers.
III. Challenges in Building a Quantum Computer:
There are several challenges associated with building a quantum computer. IBM, Google, and others are trying to make and scale up quantum computers based upon superconducting qubits. However, how to minimize the negative effect of a classical environment, which causes errors in the operation of a quantum computer, is a key problem in quantum computing.
IV. Topological Qubits:
A solution to this problem may be found in an exotic version of a qubit known as a topological qubit. Qubits based on topological superconductors are expected to be protected by the topological aspect of the superconductivity and, therefore, more robust against the destructive effects of the environment. A topological qubit relates to topology in mathematics, where a structure is undergoing physical changes such as being bent or stretched and still holds the properties of its original form. It is a theoretical type of qubit and has not been realized yet, but the basic idea is that the topological properties of certain materials can protect the quantum state from being disturbed by the classical environment.
V. Heterostructures:
The study's researchers have taken a step in this direction by developing a type of layered material called a heterostructure. The heterostructure in the study consists of a layer of a topological insulator material, bismuth antimony telluride or (Bi,Sb)2Te3, and a superconducting material layer, gallium. However, such a topological insulator/superconductor heterostructure is difficult to create due to the mismatch between the crystal structures of the two materials.
To overcome this challenge, the researchers used a technique called molecular beam epitaxy, which involves growing one atomic layer at a time to precisely control the growth of the two materials. By carefully tuning the growth conditions, they were able to create a high-quality heterostructure with a clean interface between the two layers.
The researchers then studied the properties of the heterostructure using a combination of electrical transport measurements and scanning tunneling microscopy. They found that the interface between the topological insulator and the superconductor exhibits a unique electronic state, known as a Andreev bound state, which is a signature of the proximity effect.
The proximity effect is a phenomenon where the superconducting state can penetrate into a neighboring material and induce superconductivity in that material. This effect has been observed in various types of heterostructures, but the researchers in this study were able to observe it in a topological insulator/superconductor heterostructure for the first time.
VI. Confinement Heteroepitaxy:
Another approach to creating heterostructures is called confinement heteroepitaxy. In this method, a thin film of one material is deposited onto a substrate of a different material. The substrate constrains the growth of the film, leading to the formation of a crystalline interface between the two materials. This method has been used to create a variety of heterostructures, including semiconductor/superconductor and ferromagnet/superconductor heterostructures.
VII. Research Findings:
The researchers in the study found that the heterostructure of topological insulator (Bi,Sb)2Te3 and superconductor gallium exhibited a number of interesting properties. For example, they found that the interface between the two materials was very clean, with no observable defects. They also found that the heterostructure exhibited a phenomenon called proximity-induced superconductivity, where the superconducting behavior of the gallium layer extended into the topological insulator layer.
VIII. Implications of the Research:
The development of high-quality heterostructures has many potential implications for the field of quantum computing. Heterostructures can be used to create novel materials with unique electronic and magnetic properties, and these materials may be useful for building new types of quantum devices. For example, the proximity-induced superconductivity observed in the (Bi,Sb)2Te3/gallium heterostructure could be used to create topological qubits, a type of qubit that is particularly robust against decoherence.
In addition to their potential use in quantum computing, heterostructures may have applications in other areas of technology. For example, heterostructures could be used to create new types of sensors, or to develop more efficient energy conversion devices. The development of high-quality heterostructures is therefore an area of active research, with many potential applications in a variety of fields.
IX. Conclusion:
In summary, the study's researchers have demonstrated the feasibility of creating high-quality heterostructures between topological insulators and superconductors. They have shown that such heterostructures exhibit a unique electronic state at the interface, which is a signature of the proximity effect.
These findings open up new avenues for research in the field of topological superconductivity and may have important implications for the development of future quantum technologies. By further exploring the properties of these heterostructures, researchers may be able to uncover new ways to manipulate and control the flow of electrons and ultimately realize the dream of topological quantum computing.
Comments